Metallurgy is part of the metal recycling industry. While we don’t claim to be scientists at our metal recycling Los Angeles center, an overview of and familiarity with metallurgy does come in handy. Various scientists have written extensively about the subject and the Science Direct website lists several book excerpts dealing with metallurgy. For more information you may visit their website and/or check out the books and authors delineated on the website. These include:
Stephen Hall, in Branan’s Rules of Thumb for Chemical Engineers (Fifth Edition), 2012

Common Corrosion Mistakes
Kirby gives sound advice for designers who are not experts in metallurgy. He gives seven pitfalls to avoid [8]:
1.
Not understanding details of the corrosion service. Stating only the predominant acid without the other details (such as presence of chloride ion) is an example. The author explains how to use the acronym SPORTSFAN:
S = Solvent
P = pH
O = Oxidizing potential
R = Reducing potential
T = Temperature
S = Salts in solution
F = Fluid flow conditions
A = Agitation
N = New aspects or changes to a chemical process
2.
Concentrating on overall or general corrosion, and ignoring pitting, crevice corrosion, stress corrosion, cracking, etc.
3.
Ignoring alkaline service. Just because strong alkalis do not cause severe overall corrosion in carbon steel or stainless steel, don’t overlook stress corrosion, cracking, or effects on other materials.
4.
Not considering water or dilute aqueous solutions. This can be overlooked if the other side of the tube or coil has strong chemicals, such as sulfuric acid.
5.
Confusion about L-grade of stainless steels. The L-grades, such as 304L, have lower carbon (0.03% vs. 0.08%) than the standard grades (e.g., 304). The L-grades are used to prevent “sensitization” from carbide precipitation during welding. This minimizes strong acid attack of the chromium-depleted areas along the welds. Don’t forget to specify the L-grade for the filler metal as well as the base plate. Some confusion exists that the purpose of the L-grade is to handle chloride stress corrosion cracking at percent or multiple ppm levels.
6.
Not accounting for the oxidizing or reducing potential of acidic solutions. For non-chromium-containing alloys that are capable of withstanding reducing acids, a small amount (ppm level) of oxidizing chemical can have devastating effects.
7.
Neglecting trace chemicals. Watch for ppm levels of chloride with stainless steels or ppm levels of ammonia with copper-base alloys, for example.
Introduction
Ramesh Singh, in Applied Welding Engineering (Third Edition), 2020
Metallurgy is the science and technology of metals and alloys. The study of metallurgy can be divided into three general groups:
1.
Process metallurgy
Process metallurgy is concerned with the extraction of metals from their ores and the refining of metals. A brief discussion on production of steel, castings, and aluminum is included in this section.
2.
Physical metallurgy
Physical metallurgy is concerned with the physical and mechanical properties of metals affected by composition processing and environmental conditions. A number of chapters in this section specifically address this topic.
3.
Mechanical metallurgy
Mechanical metallurgy is concerned with the response of metals to applied forces. This is addressed in subsequent chapters of this section.

Process Concept for Scaling-Up and Plant Studies
Govind S. Gupta, … S. Seetharaman, in Treatise on Process Metallurgy: Industrial Processes, 2014
3.1.4 Scaling-Up and Scaling-Down Operations in Process Metallurgy
Metallurgy used to be an art rather than science until mid of nineteenth century during which period large metallurgical plants were developed (based on empirical relations, trial and error, intuition, etc.) and operated successfully. Since more than one and a half centuries, metallurgy has emerged as a science and more predictive. Therefore, in metallurgy field, often the problems are posed in terms of real problem which plants are facing during operation like less productivity, environmental problems, etc. As these processes were evolved not based on well-established scientific criteria, therefore, to understand these processes and to address the problem, one has to scale down the industrial process (quite opposed to scaling up which is carried out more in aerospace/mechanical/chemical engineering discipline where the science is well developed and plant came up after studying the laboratory scale and pilot models) to laboratory scale. However, the principle involved in either scaling up or scaling down is the same. An excellent example of improving the process by scaling down is the study of ironmaking blast furnace. Blast furnaces used to produce 50 tons a year hot metal in late eighteenth century, and in the beginning of 21st century, its capacity has improved to more than 5.5 million tons/year. This is possible only by studying the blast furnace process in various parts to understand the science behind all the processes which are occurring in a blast furnace. To illustrate this, an example is given in section 3.1.6 on raceway size prediction as it affects the aerodynamics of the blast furnace and thus the heat and mass transfer and hence the productivity.
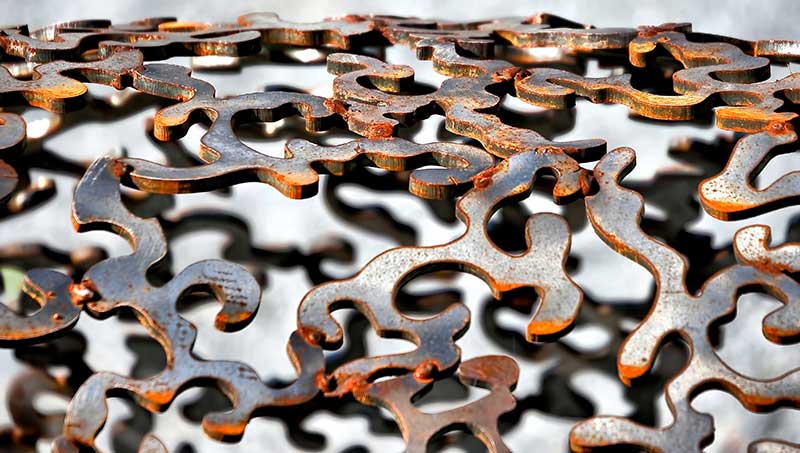
Energy consumption of the Kroll and HAMR processes for titanium production
Yang Xia, … Pei Sun, in Extractive Metallurgy of Titanium, 2020
Abstract
Metallurgy of titanium is costly, partially due to the high energy consumption during its production. In this chapter, the main determinants of the large energy consumption in the Kroll process are analyzed based on the best publicly available information. Energy consumption in the newly developed HAMR process is also modeled and analyzed. The processing energy consumption in the HAMR process is estimated to be >25% less than that of Kroll process when the regeneration of Mg is considered, while it is >65% less when the regeneration of Mg is considered separately.
Metallurgy – Corrosion
A. Kayode Coker, in Ludwig’s Applied Process Design for Chemical and Petrochemical Plants (Fourth Edition), 2015
Introduction
Metallurgy is generally dealt with by material scientists and material engineers, who study the physical and chemical behavior of metallic elements, their intermetallic compounds and their mixtures, which are referred to as alloys. Metallurgy can also be described as the technology of metals, the way in which science is applied to the production of metals and the engineering of metal components for use in products for manufacturers and consumers. The production of metals involves the processing of ores to extract the metal they contain, and the mixing of metals or other elements to produce alloys.
The use of metals often impacts the environment throughout the life cycle of the material. The supply involves the mining of the ores or minerals through processing into metals or alloys having specific composition, and manufacturing products. Lack of maintenance practice during use may result in the manufactured products causing environmental harm. Further, at the end of its active life, a metallic component may enter the solid waste stream or require energy for recycling into new material. The determined metric for environmental impact is referred to as embodied energy. The life cycle approach (LCA) is often employed to determine the energy consumption from mining, processing, etc. This involves the material from when mined to when it is ready, to be shipped to customers in the form of bulk metal. The recycle material method accounts for a certain percentage of metal being recycled, and Table 22-1 provides embodied energy estimates for some materials. Figure 22-1 shows the periodic table of the elements as an interactive periodic table including properties, compounds, isotopes.
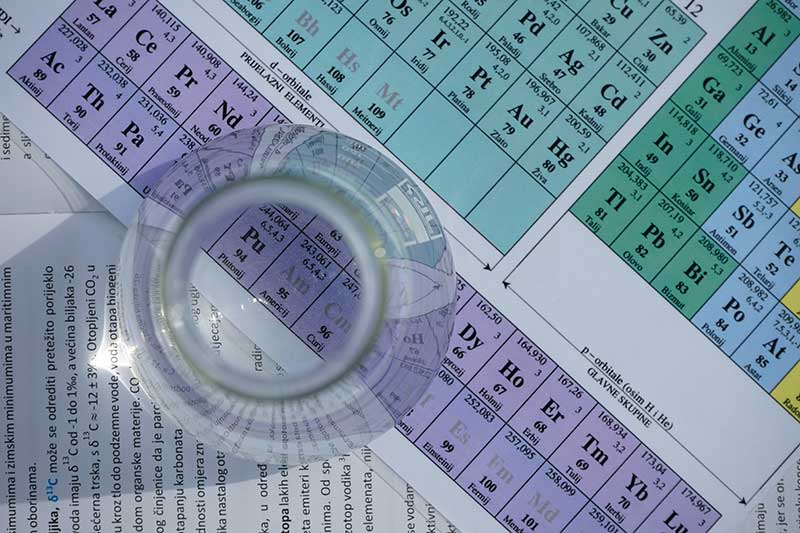
A.H. WINDLE, in Physical Metallurgy (Fourth Edition), 1996
4.1. General
Metallurgy is above all the science of alloying. From the big three: iron, copper and aluminium, and perhaps twenty other elements which are also household names, it is possible to generate more or less the complete range of alloys in common use. Of course, the interaction between pairs, or larger numbers, of elements is described, as a function of temperature and compositions, by a phase diagram. The understanding of these diagrams is as mother’s milk to a metallurgist, and their importance cannot be questioned.
Phase diagrams do exist in polymer science, but their significance is not pre-eminent, and there are many involved with polymeric materials who can ply their trade without ever having to encounter one. Why the difference?
In the first instance, the number of polymers which can be envisaged and synthesised is semi-infinite. There is a huge number of ways in which carbon, oxygen, nitrogen and hydrogen atoms can be put together to make different chains or networks. So it could be argued that instead of trying to mix different types of chains to make materials with different properties, the polymer chemist merely dreams up and synthesises another molecule. There may be something in this view; however the central reason why polymer alloys are not centre stage is that they are reluctant to form solutions or compounds with each other. They simply do not alloy very well.
The word ‘alloy’ is not used by the polymer scientist (this chapter excepted). The closest equivalent is blend. In general, solid solutions of polymers are referred to as miscible blends while two-phase mixtures where there is effectively no terminal or liquid–liquid solubility, such as in the copper-lead system, are referred to as immiscible blends.

Secondary Steelmaking
Lauri Holappa, in Treatise on Process Metallurgy: Industrial Processes, 2014
Abstract
Secondary metallurgy is a very central part of modern steelmaking process. It means a variety of different unit processes via which the final composition and even properties of steels are determined and adjusted. Typical unit processes in secondary steelmaking are deoxidation, desulfurization, degassing, decarburization, alloying and trimming additions as well as heating and temperature adjustment.
In this chapter, the fundamentals of thermodynamics and kinetics of unit processes are described. Further, the development and principles of most common technological methods to carry out processes are discussed including gas stirring, vacuum facilities, ladle furnace and chemical heating, and techniques for alloying and trimming additions.
Casting of aluminium alloys
S. Otarawanna, A.K. Dahle, in Fundamentals of Aluminium Metallurgy, 2011
Melt flow technique
Advanced thixotropic metallurgy (ATM) technique is a runner design concept developed by a group at CSIRO, Australia22. The technique employs a flow restriction section, a so-called melt pre-conditioner (MPC), in the runner. The MPC is aimed to shear and accelerate the flowing material before it reaches the gate. In HPDC, there are some equiaxed crystals, so called externally solidified crystals (ESCs), solidified in the shot sleeve prior to being injected into the die cavity. The shearing of partially solid alloy containing ESCs is believed to make ESCs smaller and more globular, and disperse porosity in the final microstructure. Due to smaller ESC size and more disperse porosity, ATM castings often show better mechanical properties than conventional ones.
Smaller and more globular ESCs decrease viscosity of the suspension and therefore reduce the tendency for cold shuts. This allows a lower gate speed than that in conventional HPDC to be used with the ATM design. Die erosion problem associated with high gate speeds can be reduced. Additional time gained from lower filling velocity enhances gas venting from the cavity. The ATM runner system is typically smaller than the conventional design, leading to better material yield and energy saving.
As we said, a basic knowledge of metallurgy is welcome when dealing with scrap metals. Los Angeles scrappers selling metals for cash need not worry about the subject because we here at TM Scrap Metals have it comvered.